Nanochemistry:
Nanotubes

Strength, Stiffness & Tenacity
Carbon nanotubes have unique mechanical properties; they are strong in the axial direction, yet relatively soft in the radial direction. They are also stiff and have high tensile strength.
The carbon atoms are in a planar honeycomb lattice, where each atom is connected to three neighboring atoms by strong chemical bonds. Due to these strong interlocking carbon-to-carbon covalent bonds, carbon nanotubes have high strength.
A pressure of 25 GPa can be applied on standard single-walled carbon nanotubes without observed deformation to the structure of the nanotube. Superhard phase nanotubes can also be synthesized, which have been demonstrated in experiments to be able to withstand the maximum pressures available from current experimental techniques (around 55 GPa). The superhard phase nanotubes will thus collapse at an unknown pressure that is greater than 55 GPa.
The bulk modulus of superhard phase nanotubes is around 462 to 546 GPa, which is even larger than that of diamond (420 GPa).
Carbon Nanotubes also have very high elastic modulus, thus they are considered as the ultimate high-strength fibers. Single-walled Nanotubes are also shown to be stiffer than steel, and are hence very resistant against damage by physical forces.
Pressure applied on one end of the tube will cause bending but will not damage the tube or cause deformations. As a result of this property, CNTs can also be used as probe tips for very high-resolution scanning probe microscopy.
Carbon nanotubes have unique thermal conduction properties, where they are very good thermal conductors along the tube axis but good insulators along the radial axis. Their thermal conduction properties are dependent on temperature and also on the large phonon mean free paths.
The strong C-C bonds, which makes carbon nanotubes exceptionally stiff and strong against axial strains, result in carbon nanotubes having very small, negligible in-plane thermal expansion but large inter-plane expansions. This is due to strong in-plane coupling as well as high flexibility against non-axial strains in carbon nanotubes.
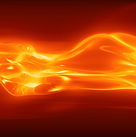
Thermal Conductivity
Properties of Carbon Nanotubes
The unique structure of Carbon Nanotubes results in unique mechanical and transport properties that allows them to be ideal fibers for various applications. In general, the properties of Carbon Nanotubes can be summarized as follows: they demonstrate a unique combination of strength, tenacity and stiffness while for other fiber materials, one or more of these desired properties are often absent. Carbon nanotubes also posses very high thermal and electrical conductivity that are similar to other conductive materials.
Toxicity and Defects
Defects
Similar to any other materials, the presence of a crystallographic defect in the structure of the carbon nanotube can significantly affect its properties. An example of a defect is atomic vacancies, where carbon atoms are missing from the hexagonal structure. In the Stone Wales defect, a missing atom causes rearrangement of the bonds form a pentagon and heptagon pair. The material's mechanical strength and tenacity is due to the strong bond in the honeycomb lattice-- as such, when defects are present at prominent levels, the tensile strength of the carbon nanotube may be lowered significantly by up to a maximum of 85%. Due to the thin, small structure of the nanotubes, the tensile strength depends on its weakest segment in a manner similar to that of a chain whose maximum strength is its weakest link.
Crystallographic defects not only affects the mechanical properties, but also the thermal and electrical properties. Conductivity is decreased through the defective region, and defects in armchair-type tubes can cause the region surrounding the defect to be semiconducting. Magnetic properties may also be induced by a single monoatomic vacancy. Phonon scattering may also occur, which will increase the relaxation rate of phonons, reducing the mean free path. This will greatly decrease the thermal conductivity of nanotubes.
Toxicity
Toxicity of carbon nanotubes has been questioned by various groups of scientists to determine the safety of its use in its various applications. Research on its toxicity began 2007, and data is still incomplete. However, preliminary results demonstrated difficulties in evaluating the toxicity of nanotubes, as its reactivity is dependent on a wide range of factors such as structure, surface area, size distribution, agglomeration state, purity, surface charge, and surface chemistry. Current available data indicates that nanotubes can pass through membrane barriers under certain conditions, hence there is a possibility of harmful effects like fibrotic reactions and inflammation if nanotubes were to reach the organs. Nanotubes may even enter cells, accumulating in the cytoplasm to cause cell death. Comparative studies in mice suggested that SWCNTs were more toxic than quartz, which is a serious health hazard when chronically inhaled.
Carbon nanotubes aligning lengthwise with the airways when deposited in the alveolar ducts and will often combine with metals. The shape of CNT fibers are also similar to the needle-like asbestos fibers. This suggests that large usage of carbon nanotubes may potentially cause pleural mesothelioma, cancer or other related diseases A recent study supports this prediction. This is of significance as research and business communities invest greatly in the use of carbon nanotubes for a variety of products as it is thought that carbon nanotubes are only as hazardous as graphite. There is hence need for more caution and further research.
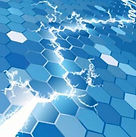
Electrical Conductivity
The electrical property (metallic or semiconducting) of carbon nanotubes canbe determined by its diameter and the amount of twist in its hexagonal, honeycomb lattice.
Electrons in carbon nanotubes can only have certain allowed energy levels, thus a nanotube is metallic if the conduction band (energy level at which delocalized electrons are allowed to flow between atoms in the nanotube) is directly above the valance band. In a metallic nanotube, electron transitions to the conduction band can occur easily, while electrons in a semiconducting nanotube do not transit as easily as the energy level of the conduction band is much higher, thus it requires additional energy, such as light for the electron transitions to occur. Armchair nanotubes has no gap between the valance bands and conduction bands, thus they are metallic. On the other hand, there is an energy gap between the valance and conduction bands in zigzag and chiral nanotubes, hence they are semiconducting.
A nanotube behaves like a ballistic conductor as the conductance of the tube is quantized. Resistance of nanotubes is also constant, and the nanotubes have been shown to have tolerance for very high current densities. Ultra-small SWNTs have also been demonstrated to exhibit superconductivity at tempperatures below 20K.
In most 3D materials, there is an absorption threshold before it experiencing an increase in absorption. However, optical absorption in carbon nanotubes have sharp peaks (1D nanotubes), thus displaying unique properties different from the conventional materials. The electrons of the carbon atoms in the nanotubes undergo electronic transitions from the v2 to c2 energy levels or v1 to c1 levels when light is absorbed. High absorbances of 0.98-0.99 can also be attained for light of far-ultraviolet (200 nm) to far-infrared (200 μm) wavelengths 0.98-0.99 by using vertically aligned "forests" of single-wall carbon nanotubes.
Photoluminiscence occurs for semiconducting single-walled carbon nanotubes, which upon photoexcitation emits near-infrared light.
However, metallic tubes do not produce flourescence. Photoexcitation of electrons still occur, but due to the availability of electrons in the metal, the holes left are instantly filled. Hence, light absorption still occurs but without formation of excitons. Photoluminiscence is also not observed in MWCNTs.
Photoluminescence, optical absorption and Raman scattering, are linearly polarized along the tube axis. In addition photoluminiscence is occurs fast as relaxation usually occurs within 100 ps. There is a wide spectral range for photoluminiscence, with emission wavelengths between 0.8 and 2.1mm. Photoluminiscence can be increased or decreased from interaction between nanotubes or with other materials.
Due to high length-to-diameter ratio, the optical properties of the nanotubes are extremely directionally dependent and tunable. It also has high selectivity in the wavelength of detection and emission of light. Thus, it is potentially useful in optics and photonics applications, such as LEDs, photo-detectors., bolometers and optoelectronic memory devices.
